Abstract
Popular discussions about nuclear power eventually get around to at least one of five objections: It's not safe; no one knows what to do about waste; it's too expensive; it leads to nuclear weapons proliferation; or there isn't enough uranium. All of these objections are baseless.
Revised to incorporate valuable comments from Dr. Yoon Il Chang, Tom Blees, and Eric Loewen.
It's not safe (yes it is)
The Paul Scherrer Institut in Villigen PSI, Switzerland is a frequent European Community consultant concerning safety. Their collection of more than 33,000 records of accidents related to electricity production shows that nuclear power is the safest-ever way to make electricity, by a very wide margin. Only 46 fatalities are directly attributed to municipal nuclear power in its entire six-decade worldwide history, all due to one accident, ironcially a botched safety test at Chernobyl.
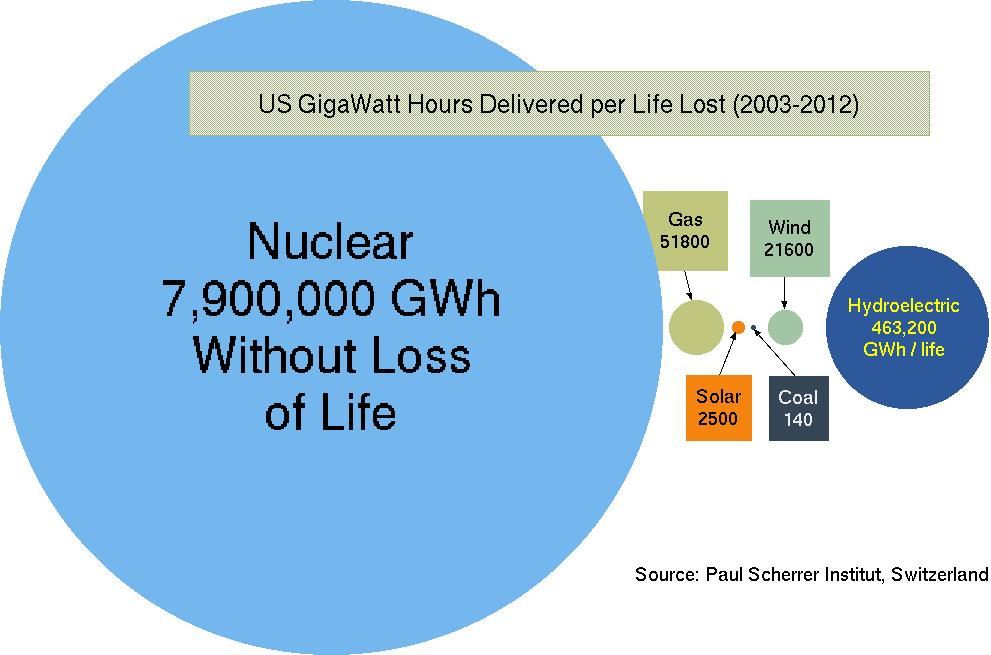
The Chernobyl reactor was the Hindenburg of nuclear reactor designs. It is irrelevant because another one with similar defects will never be built. It is described here only because of the fear it engendered.
The Soviet Union’s safety culture was different from the west’s, and reactors were not licensed because there were no licensing criteria. The Chernobyl reactor was a scaled-up version of one purpose-built to produce plutonium for weapons. As it got hotter, or if the cooling water boiled, the fission reaction ran faster, so it got hotter faster. The operators lost control of it, ironically because they bypassed the inadequate shutdown mechanisms during a safety test. The ensuing calamity was a steam explosion and a graphite fire, that dispersed several tonnes (1000 kilograms/tonne) of spent fuel near the reactor, and radioactive dust throughout the region.
The BORAX test reactor in Idaho was intentionally destroyed by prompt criticality, but licensed reactors are designed so that it is impossible to cause a nuclear explosion, either by mistake or intentionally.
The United Nations Scientific Committee for the Effects of Atomic Radiation (UNSCEAR) reported to the General Assembly in 2008 that 134 plant operators and emergency responders at Chernobyl were exposed to sufficient radiation to develop acute radiation syndrome, which caused 28 deaths. Two others died from injuries not caused by radiation (falling debris), and one from coronary thrombosis. The report noted there is “no scientific means to determine whether a particular cancer in a particular individual was or was not caused by radiation,” and there is “no scientific evidence of increases in overall cancer incidence or mortality rates or in rates of non-malignant disorders that could be related to radiation exposure.” Nonetheless, the report speculated that fifteen excess cases of fatal juvenile thyroid cancer, compared to earlier decades, out of 6,000 cases reported between 1991 and 2005, might have been caused by the accident.
In the most affected areas (Ukraine, Belarus, and southwestern Russia) the average additional radiation dose to the general public over the period 1986-2005 was about nine millisieverts (mSv). Residents “need not live in fear of serious health consequences,” according to the report.
UNSCEAR reported in October 2013 that “Japanese people receive an effective dose of radiation from normally occurring sources of, on average, about 2.1 mSv annually and a total of about 170 mSv over their lifetimes…. No radiation-related deaths or acute diseases have been observed among the workers or general public exposed to radiation from the accident…. For adults in Fukushima Prefecture, the Committee estimates [the increase in] average lifetime effective dose to be of the order of 10 mSv or less… discernible increase in cancer incidence in this population that could be attributed to radiation exposure from the accident is not expected.”
The dose from one abdominal and pelvic CT scan with and without contrast is about 30 mSv. The annual dose on the Tibetan plateau is 13-20 mSv. The annual dose on Guarapiri Beach in Brazil is 1154 mSv.
Although radioactive materials from a municipal nuclear power plant outside the Soviet Union have never caused an injury, illness, or fatality, Argonne National Laboratory (ANL) and Idaho National Laboratory (INL) believed they could develop an even safer design. During a meeting on 26 April 1944 (see Appendix B of ANL report 49168), Enrico Fermi described an energy system consisting of fast-neutron reactors with fuel reprocessing. A concept that led to the design of Experimental Breeder Reactor II, or EBR-II was described during that meeting by Leo Szilard. Leonard J. Koch became the project manager, and filled in many details during subsequent years. EBR-II was a 20 megawatt (MWe) reactor near Arco, Idaho. The Integral Fast Reactor, or IFR project, led by Charles E. Till and Yoon Il Chang, using concepts developed at EBR-II, had a goal to tie up absolutely all loose ends related to nuclear power (Although Koch had retired from ANL before the official start of IFR, his vision for the experimental program to develop it is clear in a memorandum dated September 2, 1953). An important new component was the fuel processing system that uses pyroelectric refining, instead of the melt refining method that had been used in the early years (See Chapter 8 of Plentiful Energy).
Detailed nuclear, thermodynamic, and mechanical calculations, and computer simulations, had shown that the EBR-II design was inherently safe. To demonstrate this, in 1986 Pete Planchon conducted a demonstration for an invited international audience. Automatic safety interlocks were turned off. Coolant circulation was turned off (the cause of the destruction of the Three Mile Island reactor). Core temperature rapidly increased from 1010 degrees (Fahrenheit) to 1430 degrees. Liquid sodium coolant boils at 1621 degrees. Within seven minutes the core was below operating temperature, without action by operators, computers, valves, pumps, auxiliary power, or any moving parts. The operators were not injured. The reactor was not damaged. There was no release of radioactive material. The reactor was restarted with coolant circulation restored, but the steam generator disconnected. The same scenario recurred. Two months later, the operators at Chernobyl repeated the second experiment, using a very different reactor, with tragic consequences.
The demonstrated safety of EBR-II depends only upon immutable laws of physics and thermodynamics, and the geometry and materials of the reactor core.
No one knows what to do about waste (yes we do)
Pressurized light-water power reactors produce about twenty tonnes of spent fuel per gigawatt year (GWe-year) of electricity. Spent fuel is dangerously radiotoxic for 300,000 years, an apparently intractable problem.
Examining the composition of spent fuel leads to a different conclusion. A tonne of spent fuel from contemporary light-water reactors (LWR) consists of about 52 kilograms of fission products and 948 kilograms of uranium and metals with greater atomic number, i.e., unused fuel.
Fission products are less radiotoxic than uranium in nature after 300 years, not 300,000 years.
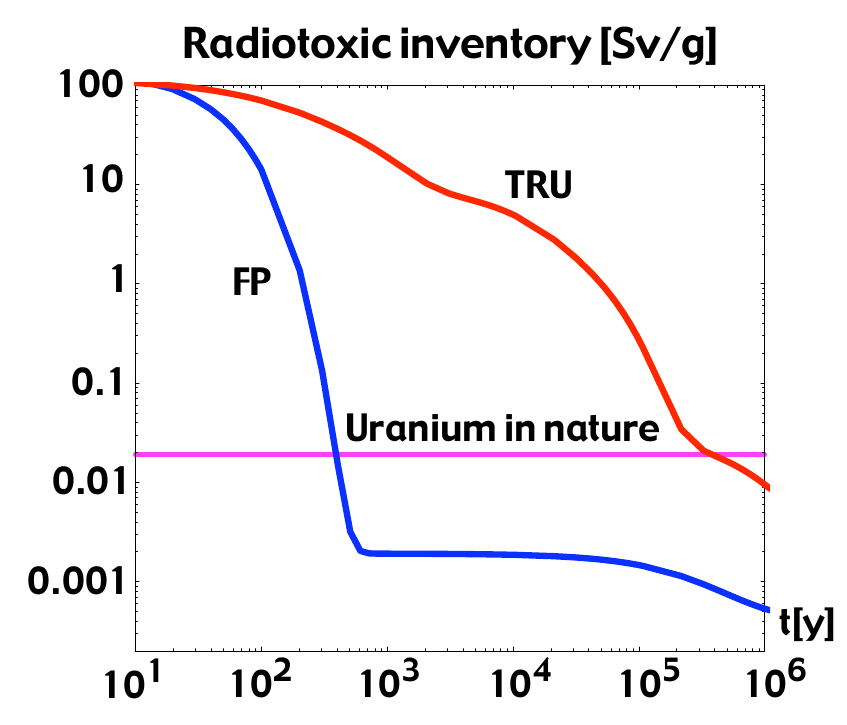
Fission products are produced at the rate of about one tonne per GWe-year. Custody of fission products separated from uranium and transuranic metals would be much simpler than for spent fuel taken as a whole. After ten years' storage, two elements, strontium and caesium, produce 99.4% of radiotoxicity, but constitute only 9.26% of the mass of fission products — five kilograms per tonne of spent fuel, or about 92 kilograms per GWe-year. 47 kilograms per tonne, or 900 kilograms per GWe-year, are low-level waste, less radiotoxic than uranium in nature, and much simpler custody is adequate.
The PUREX (Plutonium-URanium EXtraction) process separates chemically pure but isotopically mixed plutonium and uranium from spent fuel, leaving transuranic metals with the fission products. It therefore does not reduce the duration of custody. Process fluids are good moderators that efficiently adjust the average speed of neutrons into the range that causes fission. The concentration of spent fuel must be kept very low to avoid criticality accidents. A facility to process hundreds of tonnes per year occupies several thousand hectares, has several kilometers of pipes and large numbers of pumps, mixing devices, and other components, and is very expensive.
An alternative method to separate fission products from unused fuel, based upon well-known metal electrorefining methods, was developed as part of the IFR project. There is no moderator in the device, so larger amounts can be processed in a much smaller space. The pyroelectric refining device consists of an anode, composed of spent fuel, and a cathode, immersed in an electrolyte of molten lithium/potassium chloride salts.
Because of different chemical potentials, when a carefully controlled voltage is applied, uranium, transuranic metals, and active fission products such as strontium and caesium diffuse from the anode into the electrolyte and are carried through it by the electric potential gradient. Nearly pure fuel is deposited at the cathode. Active fission products remain in the electrolyte. Noble metals such as rhodium and palladium remain in the anode.
Fission products can be separated from the electrolyte by absorption into zeolyte (similar to the active material of a water softener), thereby cleansing the electrolyte for further use. Contaminated zeolyte can be mixed with powdered glass, and compressed and sintered into an impervious insoluble ceramic, ideal for storage, called sodalite. The metals remaining in the anode basket are disposed separately. This leaves the actinides.
Actinides in spent fuel consist mostly of uranium, but include significant amounts of plutonium and heavier transuranics. Odd-numbered isotopes of uranium and plutonium can be fuel in existing reactors, but even-numbered isotopes do not fission, and heavier transuranics do not fission efficiently, in light-water reactors. Using recycling, about 30% of the original fuel can be converted to fission products and electricity in an LWR, before the only slightly-smaller problem is right back where it started: A substance needing 300,000 years' custody.
If the average speed of neutrons is higher, as in IFR, all uranium and transuranic isotopes are fissionable or can be transmuted to fissionable isotopes by neutron absorption; they are fuel, not waste. Rather than 20 tonnes of intractable waste per GWe-year, a fast-neutron reactor produces one tonne of substances that require much simpler custody. Spent fuel, the substance currently and mistakenly called “nuclear waste,” of which the world is desperately eager to be rid, is effectively destroyed — and nothing else other than hideously expensive laboratory-scale toys can do it.
Fast-spectrum metal-cooled reactors should be built instead of light-water reactors because of their demonstrated safety, and their ability to destroy nuclear waste.
The first goal of the IFR project was safety. The second was to mitigate the waste “problem.”
It's too expensive (no it isn't)
A 2009 MIT study concluded that nuclear power plants could be built for $4 per watt, and produce electricity for 6¢ per kWh. Reactors under construction in Finland and Sweden cost about $7.50 per watt; ones in China cost $1.50 per watt. Delays due to lawsuits, difficulty certifying a new reactor, and licensing in an ever-changing regulatory environment add significant cost, especially interest on capital. It would be helpful if the Nuclear Regulatory Commission were to adopt the French system of licensing reactor designs, instead of individual reactors.
The operating cost of a reactor is quite low because fuel cost is low. Using $30/lb for uranium ore and 4.5% enrichment, the contribution of the cost of uranium to the price of electricity is 0.116¢ per kilowatt hour (kWh). This was the origin of Lewis Strauss’s infamous '“too cheap to meter” quip, which ignored all other costs. Reducing oxide to metal, enriching the concentration of the fissile isotope (U-235) from the natural state of 0.7%, to 5%, and fabricating fuel assemblies, increase the fuel price to 0.5¢ per kilowatt hour. Economic details are explained in Chapter 13 of Plentiful Energy.
The lowest-cost electricity in California, 5¢ per kWh, is produced by the Diablo Canyon Nuclear Generating Station. Fixed cost amortization over the life of the facility contributes 74%, or 3.7¢ per kWh. Labor and other non-fuel recurring costs are 0.8¢ per kWh. The average California delivered electricity price is 30¢ per kWh.
The 3.3 GWe Palo Verde nuclear generating station in Arizona was constructed for $1.79 per watt. Its delivered price for electricity is 4.3¢ per kWh. It is the most profitable electric utility in the U.S.
Waste disposal is incorrectly cited as a social cost not internalized in the pricing structure. Since 1981, utilities had been paying 0.1¢ per kWh into the Federal Nuclear Waste Disposal Fund for this purpose, until a Federal court ruled in 2013 they no longer needed to pay because the Department of Energy had reneged on its legal responsibility to take custody of spent fuel. It was included in the rate customers paid. The fund now stands at $43 billion. Nuclear power is the only industry that fully internalizes all costs!
Another factor sometimes cited is subsidies. Federal subsidies for light-water reactors are larger than subsidies for gas or hydro generation, but substantially less than for wind or solar photovoltaic (PV).
State and local subsidies vary. The additional California solar PV subsidy is 40% of the Federal subsidy.
The first full-scale instance of any new system is always expensive, but both construction and operating costs always decrease with experience. A 300 MWe IFR-type reactor could be built for less than $8 per watt. A GE/Hitachi consortium estimates they could build 380 MWe modular instances called S-PRISM (Super Power Reactor Innovative Small Modular) for less than $2 per watt, if they were to have a stream of orders that is sufficiently secure to justify a factory to construct essentially identical ones, instead of building each one, subtly different from any other, on site.
In Conceptual Design of a Pilot-Scale Pyroprocessing Facility, Argonne National Laboratory and Merrick & Company proposed a forty hectare $398 million pilot-scale pyroelectric refining facility to process 100 tonnes per year of any type of spent fuel, a small fraction of the cost of a PUREX facility. Operating cost would be 0.05¢/kWh. Because utilities paid into the Federal Nuclear Waste Disposal Fund, and because Yucca Mountain has been canceled, this facility and similar larger-scale facilities ought to be constructed using those funds, not funded as part of the construction of new reactors, and not from the general fund of the Federal treasury — but the Nuclear Waste Disposal Act prohibits using the funds for reprocessing.
If the goal of modernizing the energy sector is to reduce or eliminate carbon dioxide (CO2) emissions, comparison to fossil fuels is irrelevant. Several scientists calculated that the only renewable source that can in principle provide all current energy usage is solar. Wind cannot provide more than about 15% of current total energy usage, which will surely increase (and wind won't). Conservation and all other schemes, alone or together, are inadequate to close the gap between wind supply and energy demand.
Solar PV panels cost about $3 per peak installed watt of label capacity. Setting aside their inability to destroy spent nuclear fuel, it seems attention ought to focus on them instead of new designs of nuclear reactors. The amount of electricity produced in a year, divided by the amount that would be produced if the system ran continuously at full label power output, is the capacity factor. The Department of Energy reported that the 2018 national average capacity factor for solar PV was 25%. Nuclear generating stations averaged 92.5%. With a 25% capacity factor, the cost of a solar panel, at $3 per peak watt, is $12 per average watt, about six times the expected cost of S-PRISM modules.
Solar panels last about 25 years, but must operate more than four years to repay the energy invested in their fabrication, deployment, and recycling. The capital cost of $12 per average watt, amortized over twenty-five years at 5%, deducting the four-plus year energy payback period, is $26.61 per watt of average capacity.
The capital cost for solar PV panels does not include operating and maintenance costs, electricity storage, significant grid changes necessary to exploit diffuse sources, and recycling.
Several independent studies have determined that renewable sources would need 390-800 watt-hours' storage per average watt of demand to provide firm power, for which the industry definition is 99.97% availability. In Adequate Storage for Renewable Energy is Not Possible, using twelve years of data for California, I calculated that more than 2,800 watt-hours’ storage per watt of average demand would be necessary. Using five years of nationwide data, more than 800 watt-hours’ storage per watt of average demand is necessary. The May 2020 price for Tesla PowerWall 2 batteries was $0.543, not including installation. The warranty period is ten years. For 800 watt-hours, the total cost would be more than 3.8 times total USA GDP every year, for batteries alone, or “only” $49,000 per month for each of America’s 128 million households. These amounts of storage will be entirely inadequate the next time Mount Tambora erupts and produces another “year without a summer” such as 1816. This or something similar will happen again. The only question is “when?”
These sorts of calculations never appear in arguments that renewable electricity is less expensive than nuclear power.
It leads to nuclear weapons proliferation (no it doesn't)
In a March 2017 Scientific American interview, John Holdren, President Obama's Science Advisor, said “… breeder reactors… [require] what amounts to a plutonium economy… and trafficking in large quantities of weapons-usable material.”
A plutonium economy unrelated to breeder reactors already exists. The often-repeated hyperbole ”trafficking in large quantities of weapons-usable material” is nonsense.
Spent fuel from a British municipal reactor was used to make a nuclear explosion. The yield was a fraction of the Hiroshima weapon, which was a much simpler uranium device. The British remarked “We will not try that again.”
If plutonium is less than 93% isotopically and chemically pure Pu-239, explosive yield decreases rapidly. In an IFR-type system, plutonium in spent fuel never contains more than 54% Pu-239, and is never more than 40% chemically pure. Separating isotopically pure Pu-239 from spent fuel presents a much more difficult problem than for uranium. Plutonium isotopes in spent fuel, other than Pu-239, emit 50 times more heat, 5,000 times more neutrons, and 100 times more gamma radiation. This could damage a weapon or cause predetonation, and makes maintenance of fine mechanical tolerances difficult. Expensive remote assembly is mandatory. A 1994 Lawrence Livermore National Laboratory study stated “spent IFR fuel cannot be used to make a nuclear weapon without significant further processing.” No one makes weapons from spent fuel because it is the most difficult substance from which to do so.
Producing isotopically pure plutonium directly in a reactor requires controlling the neutron energy more precisely than is practical in a municipal reactor, and irradiating the fuel for durations far shorter than would be economical. Even the most rudimentary inspection regime would detect this. If an inspection regime is not practical in rogue states, don't sell them reactors, spent fuel, or means to reprocess fuel.
Even if truly “weapons-ready” material existed, the proliferation argument is a red herring. No country's nuclear power stations or fuel reprocessing affect any other country's desires, decisions, or ability to acquire nuclear weapons. On-site reprocessing implies very few opportunities for diversion or theft. Plutonium in spent fuel in an IFR-type system is in a highly-radioactive and therefore easily monitored state. Advanced industrial economies already have nuclear weapons, or have the means to make them much more effectively than from spent municipal reactor fuel. Only a fast-neutron reactor can consume all fissionable metals in spent fuel and decommissioned weapons.
There isn't enough uranium (there's plenty)
The Australian Uranium Association estimated that it is economically feasible at current prices to recover about 4.5 million tonnes of uranium. Known or projected reserves of lower quality increase the estimate to 18.5 million tonnes. Activists insist an all-electric Earth would demand about 15,000 GWe. Using the one tonne per GWe-year rule of thumb, 18.5 million tonnes is enough to satisfy this demand, if it were used with 100% efficiency, for only about 1,200 years. But today’s reactors use only 0.6% of the energy in mined uranium, so this fuel would last less than ten years. The situation isn't nearly so bleak, however.
In the United States, there are about 90,000 tonnes of 5%-used fuel, and about 900,000 tonnes of depleted uranium left over from enriching mined uranium. A 1,700 GWe all-electric U.S. energy economy could be powered by this “waste” in fast-neutron reactors for 525 years, or longer depending upon use of renewable sources, without mining, milling, refining, enriching, or importing one gram of new uranium. Spent fuel is significantly more radiotoxic than depleted uranium, so it should be consumed first. Every country that has nuclear reactors has stocks of spent fuel and depleted uranium.
IFR-type reactors extract 99.99% of the energy immanent in mined uranium but today's reactors extract only 0.6%. The price of uranium would contribute the same amount to the delivered electricity price from IFR-type reactors if it were to increase 167 fold. Uranium could be economically extracted from lower quality ores, or from seawater, where there is estimated to be at least a thousand times more than could be extracted from land. Another low-quality ore is coal-fired power plant waste, which contains nineteen times more energy in the form of uranium and thorium than was extracted by burning the coal. Thorium, four times more common than uranium, can be converted to fissile fuel by neutron transmutation in a fast-spectrum reactor.
Nuclear fission is an effectively inexhaustible source of energy.
It is possible to breed about 5% more fuel from uranium than is consumed, but only about 1% more from thorium. If the goal is to deploy a fleet of new breeder reactors fueled only by recycled fuel, thorium should not be used before sufficient reactors are in service.
The first two goals of the IFR project were safety and waste mitigation. The third was fuel economy.
The system problem
Most energy discussions focus only on components — wind turbines and solar panels.
Electricity production and distribution is a system problem, not simply a component problem.
In Burden of Proof: A comprehensive review of the feasibility of 100% renewable-electricity systems, Renewable and Sustainable Energy Reviews 76, Elsevier (2017), pp 1122-1133, Ben Heard et al described an analysis of 24 studies that claimed to explain how to construct and operate regional, national, or continental-scale electricity systems. None of the studies described systems that were physically feasible. Heard et al concluded there was no point to study economic viability.
A more serious system problem is that the Earth does not have sufficient materials to build the “technology units” that the International Energy Agency (IEA) demands be built to provide all energy from renewable sources. To stay out of the weeds, here is just one problem: Five times more copper is needed than is known to exist on the Earth in forms that can be recovered.
Additional considerations
Fast-neutron reactors can be configured to produce more fuel than they consume, which would increase the already-existing plutonium economy. Even in light-water reactors, about half of the electricity is generated by fission of plutonium created within the fuel, during operation, by neutron transmutation of otherwise non-fissile U-238. Excess plutonium from breeder reactors can be used to start additional reactors. When sufficient breeder reactors are in service, it will never again be necessary to enrich uranium. This would reduce the cost of fuel, and one who then claims to need to enrich uranium for municipal electricity service would be exposed as a liar who has a weapons program.
Plutonium is not the most toxic substance known. It is less chemotoxic than lead but dangerously radiotoxic if ingested or inhaled; yttrium-90 is 375 times more radiotoxic.
Concerns have been expressed about high-level radioactive waste shipping accidents. With on-site fuel reprocessing, there would be no need for spent fuel shipment, and only small and infrequent shipments of fissionable metals for new reactors, and fission products as impervious insoluble ceramics. At a 5% breeding rate, an IFR could breed enough fissionable material to start a new reactor every 14 years. If optimized for breeding, it might be possible to reduce the doubling time to seven years. Fifty tonnes of natural or depleted uranium, about 2.6 cubic meters, enough for a one GWe reactor's entire 50 year service life, could be delivered when the reactor is started.
The current U.S. inventory of fissionable materials is 900 tonnes in the form of spent fuel, plus 225 tonnes of weapons-grade uranium and plutonium. This is enough to start 110-140 GWe of IFR capacity, and there are no other ideas for its use. At the 5% IFR breeding rate, helped along by spent fuel from still-operating light-water reactors, the U.S. reactor fleet could reach 1,700 GWe capacity in 50-60 years without enriching any new uranium, or sooner by enriching uranium, eventually consuming the entire stock of decommissioned weapons and spent fuel — which nothing else can do.
A study commissioned by Tony Blair's government and led by Sir Nicholas Stern, former World Bank chief economist and vice president, concluded developed nations should invest 1% of GDP to reduce CO2 emissions by 25-70%, and another 1% to cope with climate change. Spending 2% of U.S. GDP during the 50-60 years required to deploy an all-IFR energy economy would cost at least $20 trillion.
Improvements to the electrical grid necessary to use dispersed and variable sources such as wind and solar would add $4-5 trillion. Storage to mitigate variability would cost more than $75 trillion, almost four times total U.S. GDP — every year!
Deploying 1,700 GWe of IFR capacity would cost $2.1-3.7 trillion, depending upon how quickly experience and economies of scale reduce costs, and would reduce net CO2 emissions by well over 95%.
Russia and France have had sodium-cooled fast-neutron breeder reactors since 1973. China has contracted to buy a BN-800 fast-neutron reactor from Russia. The BN-1200 is under development. India is developing fast-neutron breeder reactors to exploit their vast thorium reserves. The IFR program was shut down, and the EBR-II reactor was destroyed, in 1994. The Secretary of the Department of Energy, Hazel O’Leary, admitted that it cost more to shut down the project than to finish its research program. When told of this, Frank von Hippel, President Clinton’s Deputy Director of the White House Office of Science Policy replied “I know; it has to go. It’s a SYMBOL.”
Conservation, solar, wind, hydro, and minor renewable players such as tides, waves, geothermal, ocean currents, and biofuels cannot do anything to mitigate the “nuclear waste” problem.
The five oft-cited objections to nuclear power are all baseless.
It is clearly obvious that nuclear power in the form of safe fast-neutron breeder reactors with on-site pyroelectric refining must be a necessary (and economical) part of the American energy economy.
American nuclear engineers and scientists are retiring and dying faster than new ones are being prepared. America will soon be a third-world country in energy technology. Should the United States develop the technology that we invented, or buy it from France, Russia, China and India?
The sooner we start, the better off we will be.
Further reading
Van Snyder, Where Will We Get Our Energy? Amazon (2024) ISBN 979-8-884128-81-1 (hardback) 979-8-884128-67-5 (paperback) 978-1-962155-68-7 (ebook).
William Hannum, Gerald Marsh, and George Stanford, Smarter Use of Nuclear Waste, Scientific American (December 2005).
Charles E. Till and Yoon Il Chang, Plentiful Energy, Amazon (2011) ISBN 978-1466384606.
Tom Blees, Prescription for the Planet (2008) ISBN 1-4196-5582-5, ISBN-13 9781419655821.
UNSCEAR, Scientific Annex D: Health effects due to radiation from the Chernobyl accident, in Sources and Effects of Ionizing Radiation, UNSCEAR 2008 Report to the General Assembly, Volume II, ISBN-13 978-92-1-142280-1 e-ISBN 978-92-1-054482-5 (2011).
UNSCEAR, Scientific Annex A: Levels and effects of radiation exposure due to the nuclear accident after the 2011 great east-Japan earthquake and tsunami, in Sources and Effects of Ionizing Radiation, UNSCEAR 2013 Report, Volume I, ISBN 978-92-1-142291-7 e-ISBN 978-92-1-056501-1 (2014).
There are more details in my web about energy.
SEE YA ON THE NEXT FLOOR
So here's the million dollar question, who exactly is holding nuclear power down & why? I could see the O&G people not losing their business but wouldn't there still be a market for the foreseeable future? The Greenies can't all be that stupid that they can't see the benefits of small reactors next to the intended power recipients vice horribly inefficient wind & solar facilities? Did the Cuban Missile Crisis so damage the American psyche that nuclear will never be considered the preferable option?
It would seem that successive Western governments, with some notable exceptions like France, just never wanted to fully embrace the power abundance of nuclear power.
Have you seen the videos of the late nuclear chemist Galen Winsor? He was promoting nuclear power to townhalls back in the 80's. Well worth the time to watch. The links are in an article of mine:
https://www.happydiver.space/?p=1669